How far away is that? A simple question that has a lot of implications for anything in the night’s sky. As soon as you leave our own Galaxy however, it makes more sense to talk about redshift (z), the amount the light has shifted to the red due to the expansion of the Universe. In extra-galactic astronomy, this is what we use in lieu of distances.
We never express it in lightyears.
There are two major ways to determine the redshift o galaxies far far away (I mean I had to say that there). First is to take a spectrum and identify a spectral feature (bright emission lines are great for that). This allows you to measure how much that line or feature has moved to a longer (redder) wavelength very accurately. This is the best way and the most expensive way.
If the galaxies are too faint and/or there are a lot of them, getting a spectrum may not be the best option. So we use the colors of galaxies to find the redshift: the more colors, the more accurate the distance. Especially if there is a clear change in the spectrum of the galaxies — a break — allows one to get a pretty good redshifts. More colors is better, it works better for some redshifts than others. This is called photometric redshifts and they are a lot cheaper observationally since you need to take a picture anyway, why not add a few colors?
Most of the time spectroscopic and photometric redshifts agree quite well. In the paper this week, there is this check:
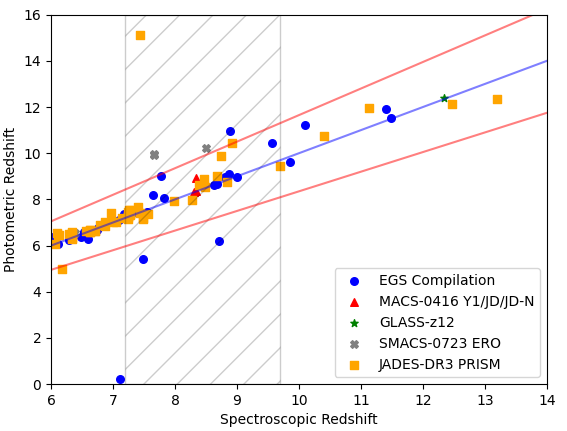
And this is the paper I am talking about that appeared this week:
We present in this paper the discovery, properties, and a catalog of 1165 high redshift $6.5 6.5$ galaxies, including…arxiv.org
This is the first of the EPOCHC papers, a project led by Chris Conselice using all the public JWST data and using them in a consistent way to get distances from the photometry. At highr redshift, the photometric redshifts get a boost from the Lyman-break, a point where all ultraviolet light is absorbed by the gas between us and the galaxy. There is light redwards of that break and none on the blue side. That sharp edge narrows the photo-z solutions nicely.
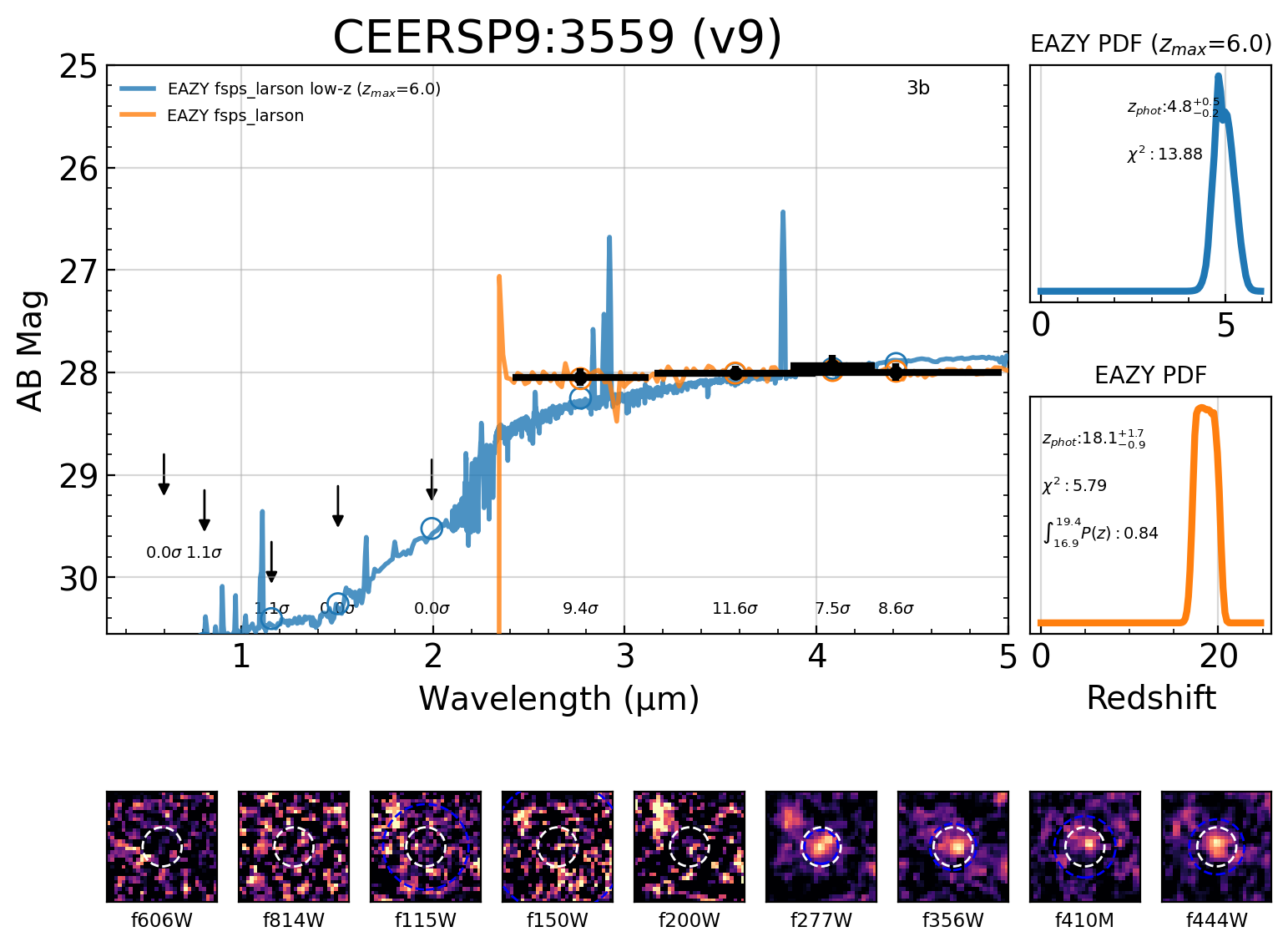
The compilation is a neat way to explore where these most distant galaxies have been found and where.

It shows very well how hard it is to get galaxies above z=10. It is still amazing we do at all! Z~10 was the limit for Hubble and we really only found one! JWST is really delivering here.
The next steps will be exploring this population of high-redshift galaxies. As you can imagine from the title, this is only the first of the EPOCHS papers with many to follow using the data compilation and redshifts presented here.
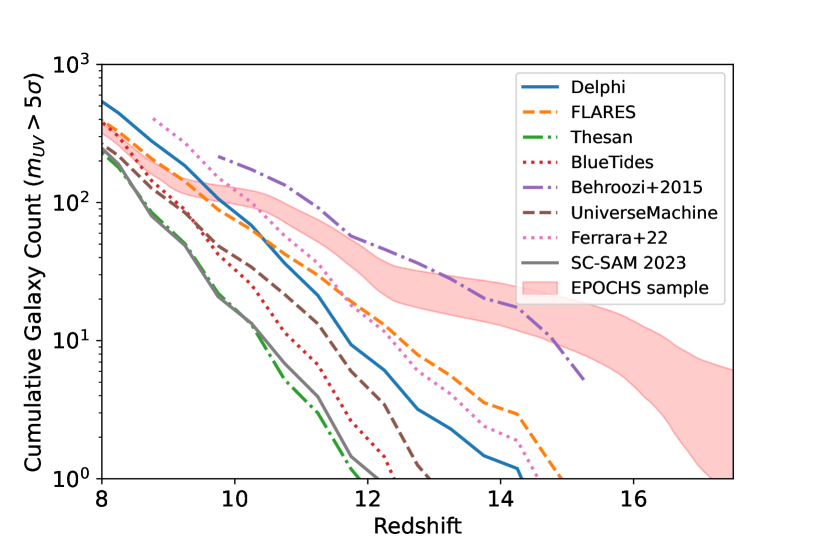
We are in trouble a little here: the models made based mostly on HST observations predicted a rapid increase of the number of galaxies. But as the figure above shows, there were many more galaxies already in place. This is causing a bit of a stir on galaxy formation models since how do you do that? There isn’t that much time to pull these galaxies together between the big bang and when we see them. The Universe assembles these fast!
It was really fun to be involved (tangentially, the main author did all the work, I shout encouragement from Kentucky as is usually my role) with this paper. I have been part of the PEARLS and CEERS teams for the past year and a half and the road of discovery, and the mode of new science is just fantastic.
Much more to come. In a next post!